Turning Black Holes into Dark Matter Labs
This video introduces a new computer simulation exploring the connection between two of the most elusive phenomena in the universe, black holes and dark matter. In the visualization, dark matter particles are gray spheres attached to shaded trails representing their motion. Redder trails indicate particles more strongly affected by the black hole's gravitation and closer to its event horizon (black sphere at center, mostly hidden by trails). The ergosphere, where all matter and light must follow the black hole's spin, is shown in teal.
Watch this video on the NASA Goddard YouTube channel.
Credit: NASA's Goddard Space Flight Center
For complete transcript, click here.
A new computer simulation tracking dark matter particles in the extreme gravity of a black hole shows that strong, potentially observable gamma-ray light can be produced. Detecting this emission would provide astronomers with a new tool for understanding both black holes and the nature of dark matter, an elusive substance accounting for most of the mass of the universe that neither reflects, absorbs nor emits light.
Jeremy Schnittman, an astrophysicist at NASA's Goddard Space Flight Center, developed a computer simulation to follow the orbits of hundreds of millions of dark matter particles, as well as the gamma rays produced when they collide, in the vicinity of a black hole. He found that some gamma rays escaped with energies far exceeding what had been previously regarded as theoretical limits.
In the simulation, dark matter takes the form of Weakly Interacting Massive Particles, or WIMPS, now widely regarded as the leading candidate class. In this model, WIMPs that crash into other WIMPs mutually annihilate and convert into gamma rays, the most energetic form of light. But these collisions are extremely rare under normal circumstances.
Over the past few years, theorists have turned to black holes as dark matter concentrators, where WIMPs can be forced together in a way that increases both the rate and energies of collisions. The concept is a variant of the Penrose process, first identified in 1969 by British astrophysicist Sir Roger Penrose as a mechanism for extracting energy from a spinning black hole. The faster it spins, the greater the potential energy gain.
In this process, all of the action takes place outside the black hole's event horizon, the boundary beyond which nothing can escape, in a flattened region called the ergosphere. Within the ergosphere, the black hole's rotation drags space-time along with it and everything is forced to move in the same direction at nearly speed of light. This creates a natural laboratory more extreme than any possible on Earth.
Previous work indicated that the maximum gamma-ray energy from the collisional version of the Penrose process was only about 1.3 times the rest mass of the annihilating particles. In addition, only a small portion of high-energy gamma rays managed to escape the ergosphere. These results suggested that a conclusive annihilation signal might never be seen from a supermassive black hole.
However, earlier work made simplifying assumptions about the locations of the highest-energy collisions. Schnittman's model instead tracks the positions and properties of hundreds of millions of randomly distributed particles as they collide and annihilate near a black hole. The new model reveals processes that produce gamma rays with much higher energies, as well as a better likelihood of escape and detection, than ever thought possible. He identified previously unrecognized trajectories where collisions produce gamma rays with a peak energy 14 times the rest mass of the annihilating particles.
The simulation tells astronomers that there is an astrophysically interesting signal they may be able to detect as gamma-ray telescopes improve.
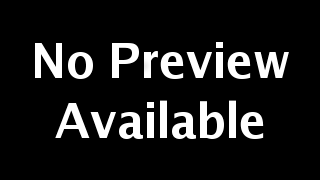
The image layers multiple frames from the visualization to increase the number of dark matter particles. The particles are shown as gray spheres attached to shaded trails representing their motion. Redder trails indicate particles more strongly affected by the black hole's gravitation and closer to its event horizon (black sphere at center, mostly hidden by trails). The ergosphere, where all matter and light must follow the black hole's spin, is shown in teal.
Credit: NASA Goddard Scientific Visualization Studio
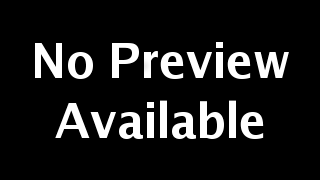
This image shows the gamma-ray signal produced in the computer simulation by annihilations of dark matter particles. Lighter colors indicate higher energies. The highest-energy gamma rays originate from the center of the crescent-shaped region at left, closest to the black hole's equator and event horizon. The gamma rays with the greatest chances of escape are produced on the side of the black hole that spins toward us. Such lopsided emission is typical for a rotating black hole.
Credit: NASA Goddard/Jeremy Schnittman
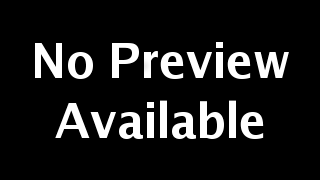
Same as above but aligned with the visualization image below.
Credit: NASA Goddard/Jeremy Schnittman
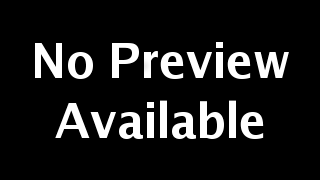
A single frame of the visulization previously described. The image is registered with the simulated gamma-ray image above.
Credit: NASA Goddard Scientific Visualization Studio
For More Information
Credits
Please give credit for this item to:
NASA's Goddard Space Flight Center. However, individual items should be credited as indicated above.
-
Producer
- Scott Wiessinger (USRA)
-
Writer
- Francis Reddy (Syneren Technologies)
-
Editor
- Scott Wiessinger (USRA)
-
Scientist
- Jeremy Schnittman (NASA/GSFC)
-
Animators
- Tom Bridgman (Global Science and Technology, Inc.)
- Scott Wiessinger (USRA)
- Chris Meaney (HTSI)
-
Videographers
- Rob Andreoli (Advocates in Manpower Management, Inc.)
- John Caldwell (Advocates in Manpower Management, Inc.)
-
Interviewee
- Jeremy Schnittman (NASA/GSFC)
Release date
This page was originally published on Tuesday, June 23, 2015.
This page was last updated on Wednesday, May 3, 2023 at 1:49 PM EDT.
Missions
This page is related to the following missions:Series
This page can be found in the following series:Tapes
The media on this page originally appeared on the following tapes:-
Dark Matter Black Hole
(ID: 2015040)
Sunday, June 14, 2015 at 4:00AM
Produced by - Robert Crippen (NASA)