Doomed Neutron Stars Create Blast of Light and Gravitational Waves
This animation captures phenomena observed over the course of nine days following the neutron star merger known as GW170817, detected on Aug. 17, 2017. They include gravitational waves (pale arcs), a near-light-speed jet that produced gamma rays (magenta), expanding debris from a kilonova that produced ultraviolet (violet), optical and infrared (blue-white to red) emission, and, once the jet directed toward us expanded into our view from Earth, X-rays (blue).
Credit: NASA's Goddard Space Flight Center/CI Lab
Music: "Exploding Skies" from Killer Tracks
Watch this video on the NASA Goddard YouTube channel.
Complete transcript available.
For the first time, NASA scientists have detected light tied to a gravitational-wave event, thanks to two merging neutron stars in the galaxy NGC 4993, located about 130 million light-years from Earth in the constellation Hydra.
Shortly after 8:41 a.m. EDT on Aug. 17, NASA's Fermi Gamma-ray Space Telescope picked up a pulse of high-energy light from a powerful explosion, which was immediately reported to astronomers around the globe as a short gamma-ray burst. The scientists at the National Science Foundation’s Laser Interferometer Gravitational-wave Observatory (LIGO) detected gravitational waves dubbed GW170817 from a pair of smashing stars tied to the gamma-ray burst, encouraging astronomers to look for the aftermath of the explosion. Shortly thereafter, the burst was detected as part of a follow-up analysis by ESA’s (European Space Agency’s) INTEGRAL satellite.
NASA's Swift, Hubble, Chandra and Spitzer missions, along with dozens of ground-based observatories, including the NASA-funded Pan-STARRS survey, later captured the fading glow of the blast's expanding debris.
Neutron stars are the crushed, leftover cores of massive stars that previously exploded as supernovas long ago. The merging stars likely had masses between 10 and 60 percent greater than that of our Sun, but they were no wider than Washington, D.C. The pair whirled around each other hundreds of times a second, producing gravitational waves at the same frequency. As they drew closer and orbited faster, the stars eventually broke apart and merged, producing both a gamma-ray burst and a rarely seen flare-up called a "kilonova."
Neutron star mergers produce a wide variety of light because the objects form a maelstrom of hot debris when they collide. Merging black holes -- the types of events LIGO and its European counterpart, Virgo, have previously seen -- very likely consume any matter around them long before they crash, so we don't expect the same kind of light show.
Within hours of the initial Fermi detection, LIGO and the Virgo detector at the European Gravitational Observatory near Pisa, Italy, greatly refined the event's position in the sky with additional analysis of gravitational wave data. Ground-based observatories then quickly located a new optical and infrared source -- the kilonova -- in NGC 4993.
To Fermi, this appeared to be a typical short gamma-ray burst, but it occurred less than one-tenth as far away as any other short burst with a known distance, making it among the faintest known. Astronomers are still trying to figure out why this burst is so odd, and how this event relates to the more luminous gamma-ray bursts seen at much greater distances.
NASA’s Swift, Hubble and Spitzer missions followed the evolution of the kilonova to better understand the composition of this slower-moving material, while Chandra searched for X-rays associated with the remains of the ultra-fast jet.
Doomed neutron stars whirl toward their demise in this illustration. Gravitational waves (pale arcs) bleed away orbital energy, causing the stars to move closer together and merge. As the stars collide, some of the debris blasts away in particle jets moving at nearly the speed of light, producing a brief burst of gamma rays.
Credit: NASA's Goddard Space Flight Center/CI Lab
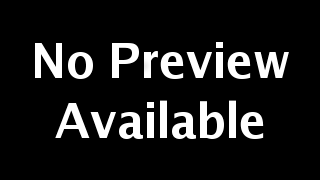
This illustration shows the hot, dense, expanding cloud of debris stripped from the neutron stars just before they collided. This cloud produces the kilonova's visible and infrared light. Within this neutron-rich debris, large quantities of some of the universe's heaviest elements were forged, including hundreds of Earth masses of gold and platinum.
Credit: NASA's Goddard Space Flight Center/CI Lab
After the neutron stars merged, the remains of the jets that produced the gamma-ray burst continue expanding into space, as shown in this illustration. After nine days, the jet directed toward us had spread laterally enough that observers could detect its X-ray emission.
Credit: NASA's Goddard Space Flight Center/CI Lab
This animation shows the shrinking orbit and explosive merging of two neutron stars, immediately followed by the eruption of powerful jets (orange) and then expanding shock waves where the jets plow into surrounding material (pink structures at the tip of each jet). The animation then shows the kilonova, the neutron-rich debris of the explosion (depicted by the expanding and flattened blue spheres) powered by the decay of newly forged radioactive elements. The jets emit gamma rays, the shock wave glows in X-rays and the kilonova produces ultraviolet light.
Credit: NASA's Goddard Space Flight Center/CI Lab
Music: "Exploding Skies" from Killer Tracks
Complete transcript available.
This animation shows the shrinking orbit and explosive merging of two neutron stars, immediately followed by the eruption of powerful jets (orange) and then expanding shock waves where the jets plow into surrounding material (pink structures at the tip of each jet). The animation then shows the kilonova, the neutron-rich debris of the explosion (depicted by the expanding and flattened blue spheres) powered by the decay of newly forged radioactive elements. The jets emit gamma rays, the shock wave glows in X-rays and the kilonova produces ultraviolet light.
This version is the raw 3840x2160, 60 fps animation and includes frames for download.
Credit: NASA's Goddard Space Flight Center/CI Lab
On Aug. 17, gravitational waves from merging neutron stars reached Earth. Just 1.7 seconds after that, NASA's Fermi saw a gamma-ray burst from the same event. Now that astronomers can combined what we can “see” (light) and what we can “hear” (gravitational waves) from the same event, our ability to understand these extreme cosmic phenomena is greatly enhanced.
Credit: NASA's Goddard Space Flight Center
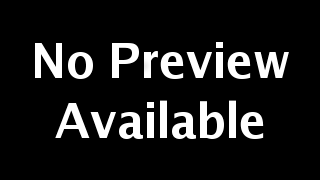
On Aug. 17, gravitational waves from merging neutron stars reached Earth. Just 1.7 seconds after that, NASA's Fermi saw a gamma-ray burst from the same event. Now that astronomers can combined what we can “see” (light) and what we can “hear” (gravitational waves) from the same event, our ability to understand these extreme cosmic phenomena is greatly enhanced. Still Image.
Credit: NASA's Goddard Space Flight Center
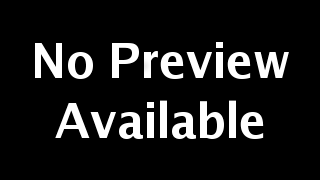
Illustration of two merging neutron stars. The rippling space-time grid represents gravitational waves that travel out from the collision, while the narrow beams show the burst of gamma rays that are shot out just seconds after the gravitational waves. Swirling clouds of material ejected from the merging stars are also depicted—these clouds glow with visible and other wavelengths of light.
Credit: National Science Foundation/LIGO/Sonoma State University/A. Simonnet
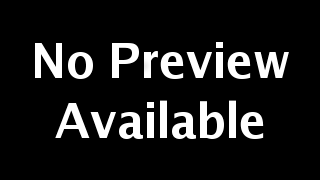
NASA's Fermi Gamma-ray Space Telescope detected a gamma-ray burst just 1.7 seconds after gravitational waves from a neutron star merger rattled Earth, confirming a long-held association. Fermi's Gamma-ray Burst Monitor, which detected the burst, sees all of the sky not blocked by Earth.
Credit: NASA's Goddard Space Flight Center
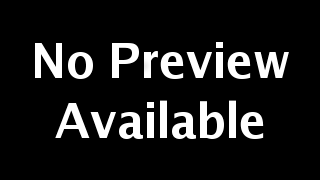
On August 17, 2017, the Laser Interferometer Gravitational-wave Observatory detected gravitational waves from a neutron star collision. Within 12 hours, observatories had identified the source of the event within the galaxy NGC 4993, shown in this Hubble Space Telescope image, and located an associated stellar flare called a kilonova (box). Inset: Hubble observed the kilonova fade over the course of six days.
Credit: NASA and ESA
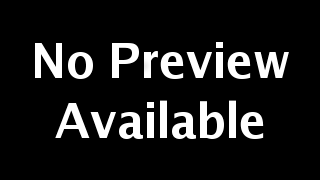
On August 17, 2017, the Laser Interferometer Gravitational-wave Observatory detected gravitational waves from a neutron star collision. Within 12 hours, observatories had identified the source of the event within the galaxy NGC 4993, shown in this Hubble Space Telescope image, and located an associated stellar flare called a kilonova. Hubble observed that flare of light fade over the course of six days.
Credit: NASA and ESA
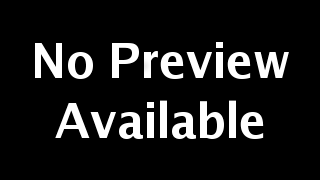
Swift’s Ultraviolet/Optical Telescope imaged the kilonova produced by merging neutron stars in the galaxy NGC 4993 (box) on Aug. 18, 2017, about 15 hours after gravitational waves and the gamma-ray burst were detected. The source was unexpectedly bright in ultraviolet light. It faded rapidly and was undetectable in UV when Swift looked again on Aug. 29. This false-color composite combines images taken through three ultraviolet filters. Inset: Magnified views of the galaxy.
Credit: NASA/Swift
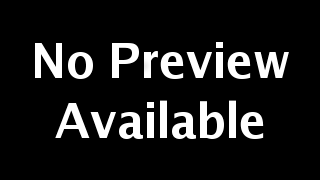
Swift's Ultraviolet/Optical Telescope imaged the kilonova produced by merging neutron stars in the galaxy NGC 4993 (box) on Aug. 18, 2017. The source was unexpectedly bright in ultraviolet light. This false-color composite combines images taken through three ultraviolet filters. Inset: A magnified view of the galaxy.
Credit: NASA/Swift
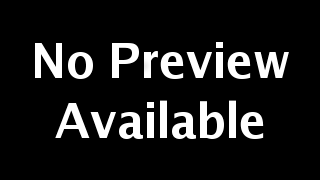
Swift's Ultraviolet/Optical Telescope imaged the kilonova produced by merging neutron stars in the galaxy NGC 4993 (box) on Aug. 18, 2017. The source was unexpectedly bright in ultraviolet light. This false-color composite combines images taken through three ultraviolet filters. Inset: A magnified view of the galaxy. No Labels.
Credit: NASA/Swift
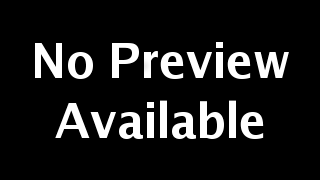
The kilonova's ultraviolet light had faded completely when Swift observed the source on Aug. 29, 2017. This false-color composite combines images taken through three ultraviolet filters. Inset: A magnified view of the galaxy.
Credit: NASA/Swift
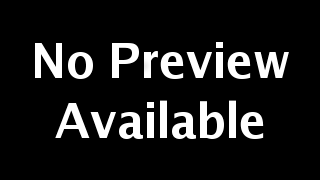
The kilonova's ultraviolet light had faded completely when Swift observed the source on Aug. 29, 2017. This false-color composite combines images taken through three ultraviolet filters. Inset: A magnified view of the galaxy. No Labels.
Credit: NASA/Swift
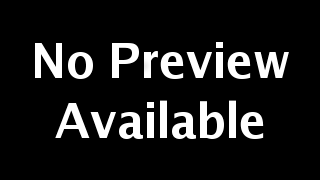
The kilonova associated with GW170817 (box) was observed by NASA's Hubble Space Telescope and Chandra X-ray Observatory. Hubble detected optical and infrared light from the hot expanding debris. The merging neutron stars produced gravitational waves and launched jets that produced a gamma-ray burst. Nine days later, Chandra detected the X-ray afterglow emitted by the jet directed toward Earth after it had spread into our line of sight.
Credit: NASA/CXC/E. Troja
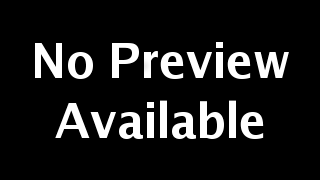
On Aug. 26, 2017, NASA's Chandra X-ray Observatory first detected X-rays from the source of the neutron star collision known as GW170817. Earlier observations did not see X-ray emission. The crash produced jets that immediately emitted a gamma-ray burst and continued on into space. Nine days later, Chandra detected the X-ray afterglow emitted by the jet directed toward Earth after it had spread into our line of sight. Labels.
Credit: NASA/CXC/E. Troja
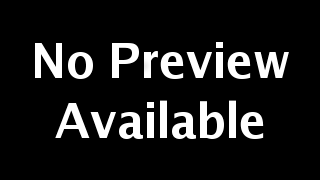
On Aug. 26, 2017, NASA's Chandra X-ray Observatory first detected X-rays from the source of the neutron star collision known as GW170817. Earlier observations did not see X-ray emission. The crash produced jets that immediately emitted a gamma-ray burst and continued on into space. Nine days later, Chandra detected the X-ray afterglow emitted by the jet directed toward Earth after it had spread into our line of sight.
Credit: NASA/CXC/E. Troja
The first sign of the Aug. 17, 2017, neutron star merger was a brief burst of gamma-rays seen by NASA's Fermi Gamma-ray Space Telescope (top). Shortly after, LIGO scientists reported detecting gravitational waves that arrived 1.7 seconds before the Fermi burst (middle). A short time later, scientists analyzing gamma-ray data from the European Space Agency's INTEGRAL spacecraft also reported seeing the burst (bottom).
Credit: NASA's Goddard Space Flight Center, Caltech/MIT/LIGO Lab and ESA
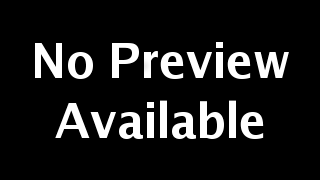
The first sign of the Aug. 17, 2017, neutron star merger was a brief burst of gamma-rays seen by NASA's Fermi Gamma-ray Space Telescope (top). Shortly after, LIGO scientists reported detecting gravitational waves that arrived 1.7 seconds before the Fermi burst (middle). A short time later, scientists analyzing gamma-ray data from the European Space Agency's INTEGRAL spacecraft also reported seeing the burst (bottom). With reporting time labels.
Credit: NASA's Goddard Space Flight Center, Caltech/MIT/LIGO Lab and ESA
On Aug. 17, 2017, gravitational waves from a neutron star merger produced a signal detected by LIGO. 1.7 seconds later, a brief burst of gamma-rays was seen by NASA's Fermi Gamma-ray Space Telescope (top). This video contains the actual sound of the LIGO detection.
Credit: NASA's Goddard Space Flight Center, Caltech/MIT/LIGO Lab
Gamma-ray bursts come from jets moving near the speed of light. How bright the burst appears depends on how we view the jet — brightest when we look straight directly down the barrel and dimmer at wider angles. This is a likely explanation for the faintness of the gamma-ray burst associated with GW170817. Since it was one-tenth as far away as similar bursts at known distances, if we viewed directly into the jet it would have appeared much brighter.
Credit: NASA's Goddard Space Flight Center
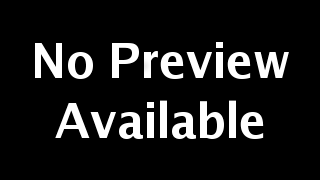
Additional still from animation. Doomed neutron stars whirl toward their demise in this illustration. Gravitational waves (pale arcs) bleed away orbital energy, causing the stars to move closer together and merge.
Credit: NASA's Goddard Space Flight Center/CI Lab
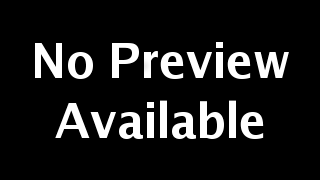
Additional still from animation. As neutron stars collide, some of the debris blasts away in particle jets moving at nearly the speed of light, producing a brief burst of gamma rays.
Credit: NASA's Goddard Space Flight Center/CI Lab
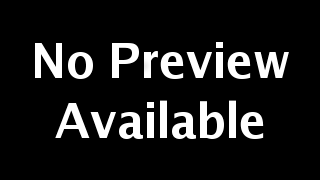
Additional still from animation. This illustration shows the hot, dense, expanding cloud of debris stripped from the neutron stars just before they collided. This cloud produces the kilonova's visible and infrared light. Within this neutron-rich debris, large quantities of some of the universe's heaviest elements were forged, including hundreds of Earth masses of gold and platinum.
Credit: NASA's Goddard Space Flight Center/CI Lab
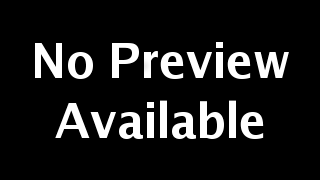
Additional still from animation. After the neutron stars merged, the remains of the jets that produced the gamma-ray burst continue expanding into space, as shown in this illustration. After nine days, the jet directed toward us had spread laterally enough that observers could detect its X-ray emission.
Credit: NASA's Goddard Space Flight Center/CI Lab
For More Information
Credits
Please give credit for this item to:
NASA's Goddard Space Flight Center. However, individual items should be credited as indicated above.
-
Producer
- Scott Wiessinger (USRA)
-
Science writer
- Francis Reddy (Syneren Technologies)
-
Animators
- Brian Monroe (USRA)
- Scott Wiessinger (USRA)
-
Scientists
- Julie McEnery (NASA/GSFC)
- Brad Cenko (NASA/GSFC)
- Eleonora Troja (University of Maryland College Park)
-
Artist
- Aurore Simonnet (Sonoma State University)
-
Graphics
- Francis Reddy (University of Maryland College Park)
Release date
This page was originally published on Monday, October 16, 2017.
This page was last updated on Thursday, October 10, 2024 at 12:17 AM EDT.
Missions
This page is related to the following missions:Series
This page can be found in the following series:Datasets used
-
[Chandra: HRC-I]
ID: 164 -
[Swift]
ID: 217 -
[Fermi]
ID: 687 -
[Hubble Space Telescope]
ID: 831
Note: While we identify the data sets used on this page, we do not store any further details, nor the data sets themselves on our site.