A 3D View of an Atmospheric River from an Earth System Model
Narrated atmospheric rivers movie
Coming soon to our YouTube channel.
Features in Earth’s atmosphere, spawned by the heat of the Sun and the rotation of the Earth, transport water and energy around the globe. Clouds and precipitation shown here are from NASA’s MERRA-2 reanalysis, a retrospective blend of a weather model and conventional and satellite observations.
Within the mid-latitudes, winds move clouds from west to east. Within the tropics easterly trade winds converge along the equator to create a moisture rich cluster of clouds, convection, and precipitation called the intertropical convergence zone, or ITCZ. Disturbances in its flow transport immense amounts of moisture and energy from the tropics to the poles. Studies have shown that atmospheric rivers account for the vast majority of the poleward transport of water vapor.
The American Meteorological Society defines an atmospheric river as “a long, narrow, and transient corridor of strong horizontal water vapor transport that is typically associated with a low-level jet stream ahead of the cold front of an extratropical cyclone.” A common measure for the strength of an atmospheric river is the integrated water vapor transport, or the amount of moisture that is moved from one place to another by the flow of the atmosphere. The blue shading shown here gives a three-dimensional view of the water vapor transport. Tropical moisture is pulled in from the ITCZ and in this example, converges with other moisture sources to form an atmospheric river. The feature then travels towards the west coast of the United States as a sub-class of atmospheric rivers commonly referred to as the “pineapple express” due to its origin near Hawai’i.
The atmospheric river is guided by the semi-permanent sub-tropical high pressure off the coast of California and the Baja Peninsula as well as the Aleutian low in the Gulf of Alaska. The pressure gradient between the clockwise flow of the Californian high and the counterclockwise flow of the Aleutian low funnel the atmospheric moisture into a narrow corridor. The more intense the pressure gradient is, the stronger the winds are that transport the water vapor. Extreme rainfall has also been associated with the more intense gradients.
Much of the moisture stays close to the surface but the rising motion of the low pressure to the north results in the air cooling, condensing the water vapor into a liquid. Precipitation over the ocean falls along the feature’s cold front on its northern side.
Another way that air can rise and condense into precipitation is through orographic lift. When air encounters the mountains along the west coast of the United States, it is forced upwards. The rising air becomes saturated, causing rain and snow to fall, particularly on the windward side of the mountain. The flow of air continues eastward, depleted of its moisture.
The precipitation that falls because of atmospheric rivers is important for the hydrologic cycle in the western United States. The winter buildup of the snowpack provides valuable freshwater resources. Despite being beneficial at times, atmospheric river induced precipitation can also be destructive. The occurrence of extreme atmospheric river precipitation events, such as the one that occurred in this example, can result in widespread flooding and mudslides.
Atmospheric rivers are not unique to the west coast of North America and occur around the globe, including Europe, New Zealand, the Middle East, Greenland, and Antarctica. The study of global phenomenon such as atmospheric rivers over the past four decades is made possible through NASA’s MERRA-2 reanalysis, a spatially and temporally consistent blend of satellite and conventional observations with a numerical model. With a dataset that provides hourly information around the globe since 1980, there is still much that can be learned about Earth’s atmosphere and the transport of water and energy around the globe.
Non-narrated version of atmospheric rivers visualization
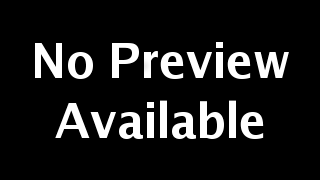
Earth surface layer color. Data on the Earth's surface is mapped in order of the color tables (snow on top and ocean on the bottom). When the data layers have very low values, that layer becomes transparent allowing the layers underneath to be seen.
Date overlay for this visualization
Credits
Please give credit for this item to:
NASA's Scientific Visualization Studio
-
Visualizers
- Greg Shirah (NASA/GSFC)
- Horace Mitchell (NASA/GSFC)
- Cindy Starr (Global Science and Technology, Inc.)
- Kel Elkins (USRA)
-
Scientists
- Allison Collow (UMBC)
- Natalie Thomas (UMBC)
- Michael Bosilovich (NASA/GSFC)
-
Producers
- Jefferson Beck (KBR Wyle Services, LLC)
- LK Ward (KBR Wyle Services, LLC)
Release date
This page was originally published on Tuesday, January 25, 2022.
This page was last updated on Sunday, October 6, 2024 at 11:05 PM EDT.
Datasets used
-
GTOPO30 Topography and Bathymetry
ID: 274 -
Winds [MERRA-2]
ID: 959 -
MERRA-2 (MERRA-2)
ID: 1134
Note: While we identify the data sets used on this page, we do not store any further details, nor the data sets themselves on our site.